In the quietude of the classroom, the teacher, a seasoned artist of words
and colors, turned to his eager student. “Imagine,” he began, “a blank
canvas or an empty page. It appears devoid of meaning, doesn’t it? But in
reality, it’s a universe waiting to be born.”
The student, puzzled, asked, “But how can something that’s empty hold
anything at all, let alone the secrets of the universe?”
The teacher smiled. “Ah, that’s the beauty of creation. The canvas, the
page, they’re not just surfaces. They’re spaces of potential. When you paint
a stroke, write a word, you’re not just adding color or letters. You’re
breathing life into an idea, giving form to the formless. You’re pulling
something out of the ether of imagination and anchoring it into reality.”
“Each stroke, each word, is like a star in the cosmos, a story in the grand
narrative of existence. And when they come together, they create
constellations of meaning, galaxies of thought. That’s how a blank canvas,
an empty page, holds the secrets of the universe. It’s a mirror reflecting
the infinite landscape of our minds, a portal connecting the realm of
thought to the world of matter.”
The student, eyes wide with newfound understanding, nodded. From that day
forward, he saw every blank page, every empty canvas, not as something void,
but as a universe brimming with possibilities, waiting to be brought to
life.
A Deep Dive into the DMC/RLB
If you’ve
examined the block diagrams for both the DMC (here
and here) and the RLB (here),
you might have noticed there’s little difference between the initial DMC diagram
from around 1996 that I posted on my site, and the more recent one from around
2015. This holds true even today. Apart from updates in circuitry and
construction methods, the DMC of the late 90's closely resembles the DMC of 2024. However,
this is not the case for the RLB. This difference arises from how each circuit
carries out its function and what is expected of
it.
To aid in understanding, I think it would be beneficial to provide a more
detailed explanation of how these two complimentary circuits work.
In a prior
discussion, I confessed my initial belief in the simplicity of harnessing
quantum entanglement to construct a Star Trek style communicator. I honestly
thought it was such an easy thing to do that someone should have already done
it. I already knew why lightspeed was the ultimate speed limit for all things,
and that it couldn’t be sidestepped. Then I learned
how quantum processes are random – truly random in nature. There was even a
word to describe how utterly random nature is: Stochastic. From my naïve
point of view, it appeared as though nature deliberately placed these hurdles
in our path to faster than light phenomena, as if to guard a valuable treasure.
Nature had
outsmarted us.
Well played, nature, well played.
This realization marked
the moment I resolved to find a way to do the impossible.
Entanglement overview
From the very beginning of my journey along this
impossible-seeming path, I knew that if any form of super-communication were
possible, Nature would be my guide. So, how does nature accomplish this miracle?
Well, we don’t know exactly how nature does her magic but we
do know what it’s called: entanglement.
Quantum entanglement is a
fascinating phenomenon that occurs when two or more particles become linked in
such a way that the state of one particle is immediately connected to the state
of other particles, no matter how far apart they are. In the case of electron spin, entanglement can occur
during certain processes, such as when a particle decays and produces an
electron and a positron. These particles have opposite spins and move away
from each other. If the spin of the electron is up, then the spin of the
positron could only be down and vice versa. This correlation holds true no
matter the distance between each particle and always occurs instantaneously.
Nature allows this correlation to occur at faster than light speeds to conserve
angular momentum.
The relevant aspect of entanglement from the point of
view of faster than light communications is that measuring the state of one
entangled particle instantly updates the state of the other. It is a continuing
and active process. So, all you need to do to send a faster than light message
is to find a way to control the spin direction of an entangled electron. To
receive the faster than light message, observe and decode the spin flips
occurring in the corresponding entangled positron. Easy-peasy. Of course, as
I’ve noted elsewhere, due to the random nature of electron spin flips, this is
impossible. Something more is needed.
Something more
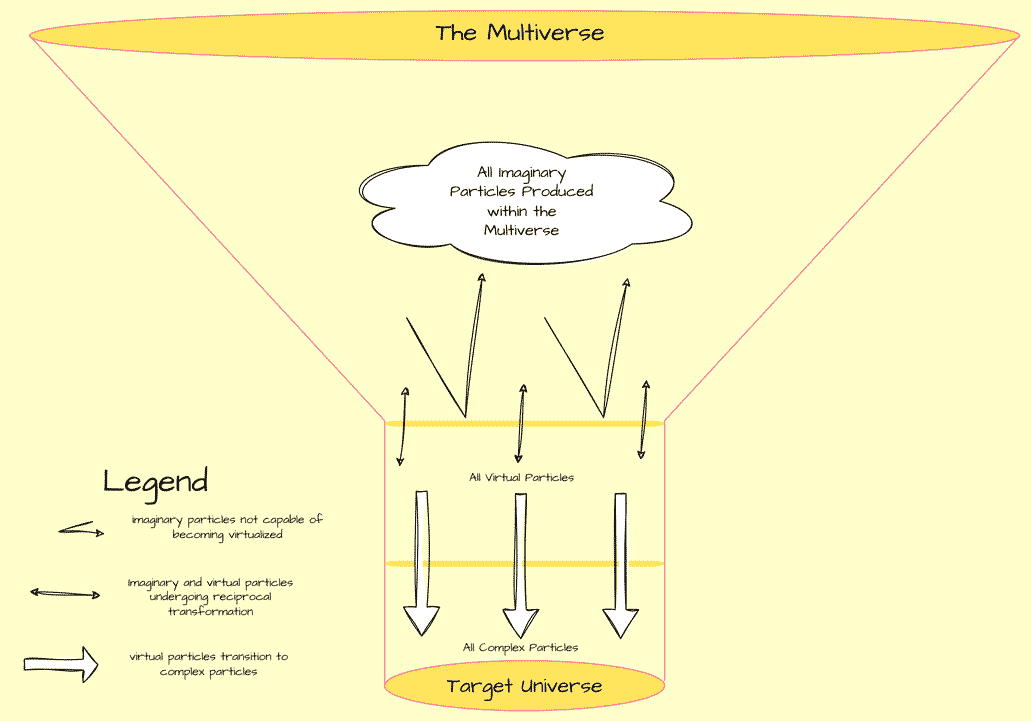
The diagram above
represents the particle hierarchy I mentioned earlier (here). Sometimes, visualizing
concepts graphically can lead to startling new insights due to their scope and
clarity. This is one such case.
The section of the graph dealing with
virtual and complex particles is straightforward. The large solid arrows
represent virtual particles that become complex with the addition of energy.
These are the particles we are familiar with, the ones we call real. Above the
domain of complex particles is the region that deals with virtual particles. The
thin double-sided arrows represent imaginary particles crossing over to become
virtual and vice versa. This part of the graph is quite straightforward.
However, the region above the virtual
particle realm led to a new insight. The long bent arrows showing deflection
represents imaginary particles that cannot cross into the virtual region of the
graph. There can be several reasons for this. First, energy considerations:
imaginary particles created as singlets, or particles with imaginary wave
functions of less than 100%, do not have the ability to become virtualized. This
also includes particles whose imaginary P2 wave function, devoid of potential,
can never collapse. Additionally, any imaginary particle whose existence in our
reality would violate physical law falls into this category. The key term here
is ‘our reality’. For example, in our reality, electrons with one-third negative
charge cannot exist. But what about another reality, one governed by a different
set of physical laws? For example, if fractional charge electrons can exist anywhere in the
multiverse, then their imaginary counterparts would exist in this region.
It took me a while to fully grasp the
implications of this new thought. The imaginary region of the graph must contain
all the particles of every universe in the entire multiverse, not just ours.
This also means that every universe in the multiverse has the potential to be
linked together through the imaginary region. I’ve often heard in discussions
about the multiverse that it would be impossible for anything to travel from one
universe to another – that this could never happen, not even in principle. I now
realized that may not be correct. All I needed to do was to find that way.
Birth of the Decision Making Circuit
I realized that the imaginary realm of particles I had postulated offered a
unique potential solution to the entanglement problem. Perhaps there existed an
alternate universe which had quantum operators that were common variables in our
universe? Perhaps there existed the equivalent of a quantum particle, call
it X, in this other universe that had the equivalent of something like the spin state
of a particle in our universe, but based
on kinetic energy and waveform - call it structure, or just 'S'. Below a certain
kinetic energy level, the S state would be describable by a sine wave, and above that
kinetic energy level, it
would be describable by a square wave. In its universe, particle structure would be
as uncontrollable as particle spin is in ours. However, in our universe,
structure S could easily be manipulated via the application of an electric field.
Faster Than Light? No Problem!
When exposed to a suitably energetic
electric field,
the X particle in one communicator would cause its entangled partner in the
other communicator to flip from sine to square, and vice-versa, regardless of distance.
Since we control the 'S' parameter, this would be how we could transmit a
digital code from point A to point B. This
communication would be faster than light, and it would actually be more
challenging to make this system work slower than light! Of course, there's that
whole problem of acquiring (or stealing) a pair of X particles from some
unsuspecting alternate universe... sigh. Why couldn't a guy ever catch a break?
The spark of creativity
Who can say where ideas, even those off-the-wall
ones, truly come from? The
process of creating something unique and worthwhile involves a complex interplay
of many factors. Imagination, knowledge, and creativity are among the most
important, but there are also factors unique to an individual, such as
education, personal history, beliefs, and desires. All these elements combine in
varying amounts within the biological melting pot we call the human brain,
giving rise to something completely unique: a single thought that will never
occur in the same way twice in all of creation.
Tesla's lightning bolt
Nikola Tesla (1856-1943), the famous
Serbian-American inventor and engineer, reportedly had his idea for the creation
of the AC induction motor while enjoying a walk with a friend in a park in
Budapest in 1882. Tesla experienced what we would call a sudden epiphany. The
plans and diagrams for the AC motor appeared like a flash of lightning in his
mind, and he feverishly scratched them out on the ground with a stick for his
friend to see. Every single alternating current motor we use today originates
from that single idea in 1882.
My gentle rainshower
Unlike Tesla’s sudden epiphany, the creation of the Decision Making
Circuit was a gradual realization that unfolded over many months of deep
contemplation. For some unknown reason, the concept of the impossible quantum
particle, X, lingered in my mind. I would slowly examine it from every angle.
Finally, one summer day in 1986, while I was sitting in the backyard, watching
fence lizards chase a cricket, everything clicked. It wasn’t a frenzied epiphany
like Tesla’s, but more like a gentle summertime rain shower. I realized that I
didn’t need to create (or steal) X; Nature had already done it for me.
The Eureka moment
I remember saying, “Damn...” and then began
sketching the diagram above, outlining the hierarchy of particle types.
By the end of the day, I had completed it. By the next weekend, I had defined
the operation of the P2 wavefunction and bounded its operation within the
imaginary realm of particles. I realized I didn’t need to steal particle X from
another universe. I didn’t even need to control the spin angular momentum of a
common particle such as an electron. All I had to do was something we already
knew how to do very well: modulate a waveform. After deconstruction, the now
imaginary waves making up that waveform would retain any information I had
put on it while it was complex. Modulated imaginary waves travel at imaginary
speeds. Of course, I didn’t know exactly what this meant. How would an imaginary
wave present itself when I finally figured out a way to receive them? Would
imaginary waves truly be instantaneous? I didn’t know, but I knew I would have a
fine time finding out, for sure!
The early years
Those early years were interesting ones. I was
building prototype DMC circuits, learning construction techniques, and making
improvements in both theory and circuitry, all without being able to receive a
signal! That was something that would not happen for another decade.
One important point I need to make here is that, in
those early years, I had yet to fully understand what the DMC really was. I had
not yet made the connection between my DMC and blackbody radiators.
physics 101: What the heck is a blackbody radiator?
So, what is a blackbody radiator? In physics, a blackbody
radiator is an idealized physical body that absorbs all incident electromagnetic
radiation, regardless of frequency or angle of incidence. Imagine a hollow
cavity with a small hole or opening in one part of its body. A photon enters the
cavity through this hole. Once inside, it is trapped, bouncing around,
reflecting off the inside walls of the cavity, or if you paint the inside of the
cavity black, it gets absorbed. It then re-emits
this energy in a characteristic spectrum that depends solely on the body’s
temperature. A perfect blackbody would let energy in via the opening but never
emit any energy at all. Ever. You can even buy blackbody radiation sources
- though they aren't perfect black body radiators, companies like CI-Systems (www.ci-systems.com)
and HGH (www.hgh-infrared.com) manufacture blackbody devices meant for testing
and calibration purposes, and are cool enough to make any science student or garage
inventor geek out.
almost perfect
There are no perfect blackbodies in nature, though some
things can come very close. We used to think a black hole was a perfect
blackbody until Stephen Hawking showed that even black holes re-emit the energy
they receive in the form of Hawking radiation. Still, of all objects that exist
in nature, black holes come the closest to being the perfect blackbody.
the missing link
Even when I finally created the first reintegration
logic block that worked, the transfer efficiency was so low—on the order of
single femtoamperes—that I didn’t make the connection for the longest time.
During that period, I was obsessed with building charge amplifiers capable of
measuring down to the one-femtoampere range. This meant that the actual usable
current involved in P2 transfer could be as low as three thousand to five
thousand electrons per second.
Such minimal values resulted in test runs
producing data nearly indistinguishable from the noise floor. It required dozens
of data sets to extract usable information and achieve even a modest efficiency.
I was so focused on the details that I missed the bigger picture entirely.
I couldn’t see the forest for the trees,
let alone the toothpicks.
I will have more to say on this in a bit.
the high school science quiz
Back in high school, towards the end of the
year, the science teacher gave us a unique quiz. Instead of the usual written
test, we were assigned to demonstrate a physical principle we had learned during
the year in front of the entire class. On the day of the quiz, students arrived
with bags full of equipment: beakers, paper, various objects, and other items
needed for their demonstrations. I, however, came empty-handed. I remember the
looks on my classmates’ faces when they saw I had nothing with me. They clearly
thought I was unprepared and that the teacher would lay into me for being lazy.
I simply shrugged and grinned.
The demonstrations begin
One by one, each student presented their
demonstration with varying degrees of success. Although it’s been a long time, I
still remember some of the impressive demonstrations. One girl demonstrated
Bernoulli’s principle by blowing air over a strip of paper, making it wave like
a flag while she explained the phenomenon. Another boy showed how current moving
through a wire creates a magnetic field by using a coil of wire connected to a
battery to move a compass needle.
My turn
Finally, it was my turn. I walked up and calmly
announced that I was going to generate a gravity wave approximately 46,500 miles
long. I saw some of those young eyes staring at me widen when I told them the
length of my gravity waves. Even Stewart, who was happy he was getting a D in
the class, knew our lab wasn't that big... I then extended my arm and waved it up and down four times a second. The
teacher asked me what the amplitude of my gravity wave was, and I promptly
replied, “One foot peak to peak.” Then I returned to my seat. I received credit
for my answer, though the instructor told me after class that it would have been
more impressive if I had demonstrated a gravity wave detector instead.
Stewart's reaction
After
class, in the hallway, Stewart who had demonstrated sound waves moving through
air by loudly farting, told me he wished he had thought of my idea instead
because his demo only resulted in a note home...ahhh, Stewart... I wonder if
he's still alive...
the bigger picture
Other than being a nice story, why am I telling you this? Because of how
it relates to the subject we are discussing. Incredibly weak though they are,
any moving mass, like my arm in the above example, generates gravity waves. Any
form of motion also generates QTEs, from which all P2 waves originate. Like
those gravity waves I generated as a teenager, P2 waves are also incredibly easy
to create. All you need to do is move something - charge, mass, particles,
anything at all. Though I didn’t know it at the time, I was also generating P2
waves right along with those gravity waves. Also, like gravitational radiation,
P2 waves are much more difficult to detect than to create. The teacher’s wry
comment concerning the gravity wave detector could apply equally well to the P2
wave detector, the reintegration logic block.
